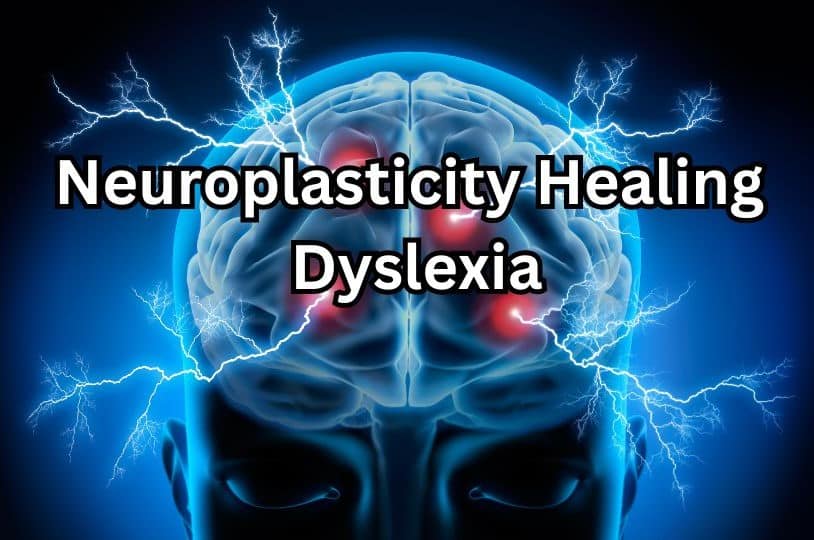
Neuroplasticity or brain plasticity refers to the brain’s ability to change in response to learning. Learn how neuroplasticity, together with cognitive training, reading intervention, and proper learning principles, holds the answer to healing dyslexia.
We specialize in helping children overcome the symptoms of dyslexia. Book a free consultation to discuss your child’s learning needs.
.
Table of contents:
- What is neuroplasticity?
- The brain cannot change
- Brain plasticity is discovered
- How the nervous system works
- The brains of people with dyslexia
- Reduced plasticity in the dyslexic brain
- What does it mean?
What is neuroplasticity?
Neuroplasticity is one of the most extraordinary discoveries of the 20th century. It showed that our brains are not fixed once we grow up.
New brain cells are constantly being born and dying, new connections form and the internal structures of the existing synapses change. When a person becomes skilled in a specific domain, the brain areas involved in that skill grow. Neuroplasticity allows brain cells to adapt to injury and disease and change their activities in response to new situations or environmental changes.
The brain cannot change
For centuries, it was proclaimed that the brain could not change, let alone improve. People were told that when something was wrong with their brain, it could not be fixed. Experts believed that humans were born with all their brain cells, lost brain cells daily, and that our brains did not generate or replace lost cells with new ones.
The belief that the brain could not change had three primary sources (Doidge, 2007):
- Brain-damaged patients rarely made full recoveries
- We couldn’t see the living brain’s microscopic activities
- The idea that the brain is like a magnificent machine, a belief dating back to the early days of modern science
And while machines do many extraordinary things, they do not change and grow. The brain was seen as a complicated machine with fixed limits on memory, processing speed, and intelligence.
Today, we know the brain can change its structure and function, thereby increasing its learning capacity. If certain “parts” fail, other parts can sometimes take over. Plasticity exists from the cradle to the grave.
Neuro stands for “neuron,” the nerve cells in our brains and nervous systems. Plastic stands for “changeable, malleable, modifiable.” Scientists initially hesitated to use the term “neuroplasticity” in their work, and their colleagues criticized them for advocating a fanciful idea. However, they persisted and gradually overturned the belief in the unchangeable brain.
One daring scientist was James Hinshelwood, today considered the father of the study of dyslexia. Hinshelwood believed the brain to be plastic and used the word brain plasticity in his classic book, Congenital Word-blindness, published in 1917.
Hinshelwood considered dyslexia (at the time called word-blindness) to be treatable through personal and systematic instruction. Not only did he have hope for children with developmental dyslexia but also for people who acquired dyslexia later in life due to disease or brain damage. Hinshelwood believed the brain could be reeducated so that the corresponding center on the opposite side of the brain would take over the functions of the defective cerebral area, and he detailed case studies of his patients who had done so.
Unfortunately, in the decades to follow, most scientists continued to maintain that the brain cannot change. They believed that if a part of the brain got damaged due to an injury or if a person was born with a mental condition, nothing could be done (Keshav, 2018). This belief left little hope for people with dyslexia!
Brain plasticity is discovered
A significant study published in 1998 established that the human brain can create new brain cells. The study challenged the widely accepted belief that the human brain was a fixed system unable to generate new brain cells (Eriksson et al., 1998).
Further research showed that the brain is flexible and adaptable, also known as “plastic.” In a classic experiment from 2000, researchers compared the brains of London taxi drivers with those of non-taxi drivers, and in 2006, they also compared them with the brains of bus drivers. These two studies demonstrated that the brain can grow.
Neuroscientist Eleanor Maguire from UCL decided to study London cab drivers after researching the memory champions in the animal world. Some birds and mammals, like western scrub jays and squirrels, store food and later retrieve it, which requires them to remember the locations of all their hiding spots. Researchers observed that a part of the brain called the hippocampus, critical for long-term memory and spatial navigation, was significantly larger in these animals compared to similar species that did not store their food. In some species, the size of the hippocampus increases during seasons when their spatial ability is most needed.
Maguire wondered if the hippocampus would grow in people who had to memorize many visual locations, like London cab drivers who memorize about 25,000 city streets and thousands of tourist attractions and hot spots before getting their cab licenses (Maguire et al., 2000).
Maguire’s studies found that London taxi drivers had larger posterior hippocampi than regular people (Maguire et al., 2000) and London bus drivers (Maguire et al., 2006). The reason is that taxi drivers need to navigate through different routes around the city, while bus drivers follow a limited, fixed set of routes. Additionally, the more years of navigation experience a taxi driver had, the larger their hippocampal gray matter volume.
Similar findings of environmentally driven plasticity have been reported in other groups, including musicians, jugglers, and bilinguals (Munte et al., 2002; Gaser & Schlaug, 2003; Draganski et al., 2004; Mechelli et al., 2004). For example, professional musicians have more gray matter in certain brain areas the longer they practice and play (Gaser & Schlaug, 2003; Sluming et al., 2002). The same is true of early bilinguals (Mechelli et al., 2004).
A study led by UCL found that professional foot painters develop ‘hand-like’ maps of their toes in their brains. (Dempsey-Jones et al., 2019). Skeide et al. (2016) discovered that when adults learn to read for the first time, changes occur not only in the outer layer of the brain (cortex) but also in deep brain structures like the thalamus and brainstem. This study observed illiterate Indian women who learned to read and write over six months.
It is now believed that a brain can rewire itself after an injury. The healthy parts of a damaged brain can be trained to take over the functions of the damaged tissue. Hinshelwood expressed this idea in 1917, which is now supported by various studies (Kadosh & Walsh, 2006; Nudo, 2013).
How the nervous system works
Neurons, also known as nerve cells, are the basic units of the brain and nervous system. They receive sensory input from the outside world, send commands to our muscles, and transmit electrical signals at every step in between.
The nervous system has two parts. The first part is the central nervous system, which includes the brain and spinal cord. This part acts as the command-and-control center of the nervous system and was believed to lack plasticity. The peripheral nervous system is the second part. It carries messages from the sense receptors to the spinal cord and brain and from the brain and spinal cord to the muscles and glands. The peripheral nervous system was long known to be plastic. For example, if one cuts a nerve in one’s hand, it can “regenerate” or heal itself.
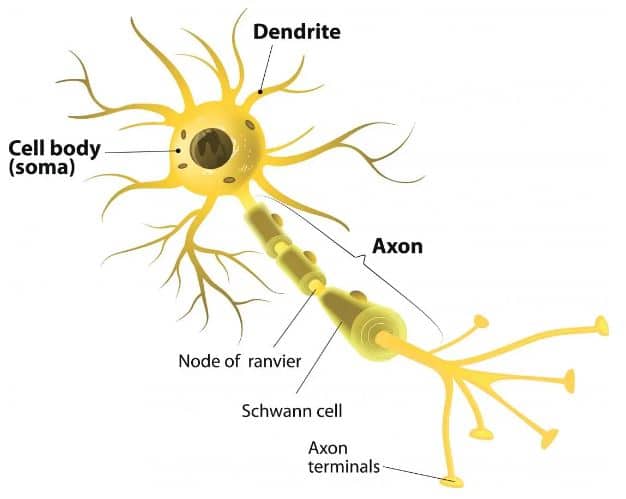
Each nerve cell has three parts: dendrites, a cell body (soma), and an axon. The dendrites are like tree branches that receive input from other nerve cells. These dendrites lead into the cell body, keeping it alive and holding its DNA. Finally, the axon is a living cable of varying lengths — from very tiny lengths in the brain to some that run down to the legs and reach up to six feet long. Axons carry electrical signals at high speeds, from 2 to 200 miles per hour, toward the dendrites of neighboring nerve cells.
A nerve cell receives two signals from other nerve cells: those that excite it and those that inhibit it. When a nerve cell gets enough excitatory signals, it will send out its own signal. On the other hand, when it gets enough inhibitory signals, it becomes less likely to send a signal. The space between the axon and the neighboring dendrites is called a synapse. Once an electrical signal reaches the axon’s end, it triggers the release of a chemical messenger, called a neurotransmitter, into the synapse. This chemical messenger then moves to the dendrite of the adjacent nerve cell, either exciting or inhibiting it.
When we talk about nerve cells “rewiring” themselves, we mean that changes happen at the synapse, either strengthening and increasing the connections between nerve cells or weakening and decreasing them.
The brains of people with dyslexia
Already in the late 19th century, Berlin (Opp, 1994), Morgan (1896), and Hinshelwood (1895) linked dyslexia to brain functioning or brain lesions. In the 1980s and 90s, autopsy studies of individuals with known dyslexia histories appeared to support these long-standing beliefs.
As technology advanced, neuroscience increasingly contributed to dyslexia research. Studies have confirmed that people with dyslexia have distinct brain differences in structure and function compared to typical readers.
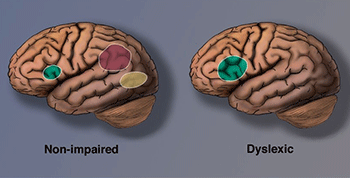
The most consistent finding concerns the left occipitotemporal cortex (shown in yellow), which includes the so-called visual word form area (VWFA), which is critical for reading (Glezer et al., 2016). In skilled adult readers, this area groups the letters of words into visual units, allowing the reader to recognize words without having to scan the individual letters.
Another part of the brain involved in reading is the left inferior parietal lobe (shown in red). It helps with analyzing words, converting letters to sounds, and processing both the sounds and meanings of words.
Both the VWFA and the parietal lobe may be impaired in students with dyslexia, which can lead to overactivation in other parts of the reading system (shown in green). When the VWFA is affected, children may read slowly and lack fluency. When the parietal lobe is affected, students will have trouble sounding out new words and may be limited to reading familiar words and guessing at unfamiliar ones.
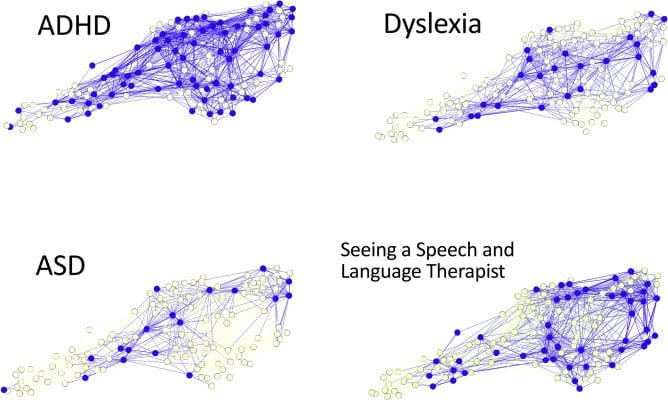
In contrast, researchers at the University of Cambridge found no specific brain areas that cause learning disabilities like dyslexia (Siugzdaite et al., 2020). Instead, they discovered that children’s brains are organized around hubs, just like an efficient traffic system or social network. Children with well-connected brain hubs had no cognitive difficulties or specific cognitive difficulties, such as poor listening skills. On the other hand, children with poorly connected hubs had widespread and severe cognitive problems, just like a train station with few or poor connections.
It is not clear whether brain differences cause dyslexia or are a result of it. For example, Krafnick et al. (2014) concluded that the brain differences found in the left-hemisphere language-processing areas seem to be a result of reading experiences rather than a cause of dyslexia.
Reduced plasticity in the dyslexic brain
So far, things seem simple and straightforward: the brain can change, so let us use neuroplasticity — which underpins the ability to learn new things — to heal dyslexia. Unfortunately, there is a snag. Research conducted by neuroscientists at MIT found that the brain’s ability to change is reduced in individuals with dyslexia (Perrachione et al., 2016).
The MIT team used MRI scans to study the brains of young adults with and without dyslexia as they did different tasks. In the first part of the study, the participants listened to a series of words read by either a single speaker or four different speakers.
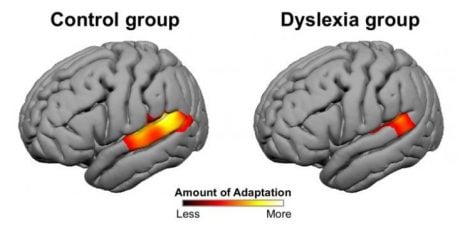
In people without dyslexia, parts of the brain involved in language showed neural adaption (change) after hearing words said by the same person but not when different people said the words. However, people with dyslexia showed much less adaptation when hearing words said by a single person.
Next, subjects were asked to look at the same or different words, pictures of the same or different objects, and pictures of the same or different faces. The researchers found that in people with dyslexia, the brain regions responsible for interpreting words, objects, and faces showed significantly reduced adaptation compared to controls when the same stimuli were repeated multiple times.
Because adaptation is also decreased for the nonlinguistic categories of visual objects and faces, the researchers concluded that dysfunction of neural adaptation is not about reading per se but a broad difference in perceptual learning.
In their final experiment, the researchers tested first and second-grade students with and without dyslexia. They found the same disparity in neural adaptation.
What does it mean?
What does the MIT team’s research mean for people with dyslexia? Does it suggest that memorization and rote learning are ineffective learning strategies for dyslexia? Does it imply that dyslexia cannot be remedied? Were Clark and Gosnell correct when they stated that “dyslexia is like alcoholism … it can never be cured” (1982, pp. 55-56). Or is there hope? Was James Hinshelwood correct in believing that dyslexia can be turned around?
Once thought of as a rigid system, we now know that the brain is plastic. In fact, it is a powerhouse. It has enabled humans to reach the moon, developed the silicon chip that can perform billions of calculations per second, created traffic lights that manage millions of people daily, and even found ways to examine its own inner workings. Is this fantastic organ able to heal learning obstacles like dyslexia despite apparent brain differences?
Before continuing to Part 2 of this article, watch our playlist of customer reviews and experience how Edublox training and tutoring help turn dyslexia around.
Edublox offers cognitive training and live online tutoring to students with dyslexia, dysgraphia, dyscalculia, and other learning disabilities. Our students are in the United States, Canada, Australia, and elsewhere. Book a free consultation to discuss your child’s learning needs.
Authored by Sue du Plessis (B.A. Hons Psychology; B.D.), an educational and reading specialist with 30+ years of experience in the learning disabilities field.
References:
Clark, M., & Gosnell, M. (1982, March 22). Dealing with dyslexia. Newsweek, 55-6.
Dempsey-Jones, H., Wesselink, D. B., Friedman, J., & Makin, T. R. (2019). Organized toe maps in extreme foot users. Cell Reports, 28(11): 2748 Doi: 10.1016/j.celrep.2019.08.027
Doidge, N. (2007). The brain that changes itself. Penguin Publishing Group.
Draganski, B., Gaser, C., Busch, V., Schuierer, G., Bogdahn, U., & May, A. (2004). Neuroplasticity: Changes in grey matter induced by training. Nature, 427: 311-2.
Eriksson, P. S., Perfilieva, E., Björk-Eriksson, T., Alborn, A., Nordborg, C., Peterson, D. A., Gage, F. H. (1998). Neurogenesis in the adult human hippocampus. Natural Medicine, 4(11): 1313-7.
Gaser, C., & Schlaug, G. (2003). Gray matter differences between musicians and nonmusicians. Annals of the New York Academy of Sciences, 999: 514-7.
Glezer, L. S., et al. (2016). Uncovering phonological and orthographic selectivity across the reading network using fMRI-RA. Neuroimage, 138: 248-256.
Hinshelwood, J. (1895). Word-blindness and visual memory. Lancet, 146(3773): 1564-70.
Hinshelwood, J. (1917). Congenital word-blindness. London: Lewis.
Kadosh, R. C., & Walsh, V. (2006). Cognitive neuroscience: Rewired or crosswired brains? Current Biology, 16(22), R962-3.
Keshav, M. (2018). The life transforming power of NLP. Chennai: Notion Press.
Krafnick, A. J., Flowers, D. L., Luetje, M. M., Napoliello, E. M., & Eden, G. F. (2014). An investigation into the origin of anatomical differences in dyslexia. Journal of Neuroscience, 34(3).
Maguire, E. A., Gadian, D. G., Johnsrude, I. S., Good, C. D., Ashburner, J., Frackowiak, R. S. J., & Frith, C. D. (2000). Navigation-related structural change in the hippocampi of taxi drivers. Proceedings of the National Academy of Sciences of the United States of America, 97(8): 4398-403.
Maguire, E. A., Woollett, K., & Spiers, H. J. (2006). London taxi drivers and bus drivers: A structural MRI and neuropsychological analysis London taxi drivers and bus drivers. Hippocampus, 16(12): 1091-101.
Mechelli, A., Price, C. J., Friston, K. J., & Ashburner, J. (2005). Voxel-based morphometry of the human brain: Methods and applications. Current Medical Imaging Reviews, 1: 105-13.
Morgan, W. P. (1896). A case of congenital word blindness. The Lancet, 2(1871): 1378.
Munte, T. F., Altenmuller, E., & Jancke, L. (2002). The musician’s brain as a model of neuroplasticity. Nature Reviews Neuroscience, 3(6): 473-8.
Nudo, R. J. (2013). Recovery after brain injury: Mechanisms and principles. Frontiers in Human Neuroscience, 7(887). Doi: 10.3389/fnhum.2013.00887
Opp, G. (1994). Historical roots of the field of learning disabilities: Historical roots of the field of learning disabilities: Some nineteenth-century German contributions. Journal of Learning Disabilities, 27: 10-9.
Perrachione, T. K. , Del Tufo, S. N., Winter, R., Murtagh, J., Cyr, A., Chang, P., … Gabrieli, J. D. E. (2016). Dysfunction of rapid neural adaptation in dyslexia. Neuron, 92(6): 1383-97.
Siugzdaite, R., Bathelt, J., Holmes, J., & Astle, D. E. (2020). Transdiagnostic brain mapping in developmental disorders. Current Biology, 30(7).
Skeide, M. A., Kumar, M., Mishra, R. K., Tripathi, V. N., Guleria, A., Singh, J.P., … & Huettig, F. (2017). Learning to read alters cortico-subcortical cross-talk in the visual system of illiterates. Science Advances, 3(5).
Sluming, V., Barrick, T., Howard, M., Cezayirli, E., Mayes, A., & Roberts, N. (2002). Voxel-based morphometry reveals increased gray matter density in Broca’s area in male symphony orchestra musicians. Neuroimage, 17(3): 1613–22.